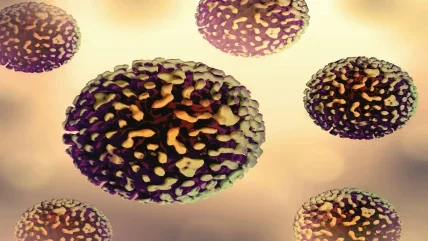
The immune system is the body’s defence against infections of all types. The first level of this system eliminates numerous pathogens before they have a chance to reach tissues or blood, through the use of the skin barrier, tears, sweat and the mucosal linings of the nose and lungs. But if a pathogen reaches the next level, a system of cells and proteins identify it and launch a specific attack to prevent it from spreading. In the case of sepsis, this immune response is impaired. “Sepsis is a systemic infection that leads to a body-wide inflammatory response that causes multiple organ Kateryna failure and death,” explains Donald Ingber, founding director of Harvard University’s Wyss Institute for Biologically Inspired Engineering. According to the WHO, sepsis is responsible for one in five deaths worldwide. What makes the disease so deadly is the rate at which it progresses, coupled with the time it takes to diagnose and treat it, with studies estimating that the chance of sepsis progressing to severe sepsis and septic shock, causing death, rises by 4% to 9% for every hour treatment is delayed. “It’s caused by infection, but you often don’t know what the cause of that infection is,” says Ingber. This, he adds, is because the way in which most pathogens are detected is using the “75-year old technology” that is blood cultures, which means “it takes days before you get results”. Molecular diagnostic methods like PCR and mass spectrometry can identify pathogens more quickly, but Ingber says these are expensive for hospitals and they can still take “a day or so” because the pathogen must be multiplied for detection to be accurate, which is too long when every hour brings patients closer to death.
For reasons still unknown to the world of medical research, the progression of sepsis happens even quicker in children, with 40% of all sepsis deaths occurring in children under five years old, according to the WHO. The current standard of care tends to be the use of broadspectrum antibiotics but, in many cases, the pathogen is never detected and so they miss the target. That’s why Ingber and his colleague Michael Super, senior staff scientist at the Wyss Institute, have been on a journey spanning more than a decade to create a new technology designed to shorten the time from diagnosis to treatment dramatically through broad-spectrum pathogen capture.
Creating FcMBL
The technology evolved from experiments performed by Ingber using microfluidics about a decade and a half earlier. “These were tiny devices with hollow channels, and what’s interesting about them is that you can have two little channels coming together like two tributaries into a river,” he says. “If you have a red and yellow dye, they go right by each other. That made me think ‘well, maybe we could have blood and sterile saline and figure out a way to pull pathogens from them, discard them, and cleansed blood would go back to the patient like with a dialysis machine’.” He put it to the test using magnetic beads coated with different antibodies and very quickly figured out what didn’t work, but was still on the hunt for an antibody that did, which is where Super came into the equation. “During my PhD thesis I co-discovered what we now call human mannose binding lectin, or MBL,” says Super. “MBL is a key component of the innate immune system, which is the immediate early response to infection.”
Super explains that, in essence, MBL is the body’s way of determining what is host and what is foreign and determining how to prevent the foreign invader from coming into the body, or if it does, how best to attack it. It does this by binding to the sugars on the surface of pathogens and releasing chemical signals that start “a feedback loop of amplification” which results in “a massive shower” of MBL on the pathogen, explains Super, which is what phagocytes, a type of white blood cell designed to engulf and kill invaders, use to identify them as foreign.
“During my PhD thesis I co-discovered what we now call human mannose binding lectin. It is a key component of the innate immune system, which is the immediate early response to infection.”
Michael Super, senior staff scientist at the Wyss Institute
To make MBL a viable choice for pathogen capture, Super and Ingber first had to overcome two challenges: producing it at a low enough cost that it was viable in the clinic, and preventing it from activating the immune system in a way that was known to cause cardiovascular inflammation. Super tackled both by creating FcMBL, a fusion of the tail region of the human IgG1 antibody with MBL. Tests comparing the binding behaviours of both MBL and FcMBL showed no difference between the two, other than the fact that FcMBL had to be applied to magnetic beads like in Ingber’s earlier experiments.
Military funding
The studies published by Ingber and Super caught the attention of Darpa, the research and development agency of the United States Department of Defense responsible for the development of emerging technologies for use by the military. “Companies had been developing portable dialysis units and the military had been deploying them in warzones,” says Ingber. “They were very interested in leveraging [our work] to develop sepsis therapy. That’s where a lot of our initial funding came from.” The original design using magnetic beads wasn’t viable for a device that had to be produced at scale, so Ingber and Super applied the FcMBL directly to a dialysis-like filter, and in doing so created a treatment for sepsis that’s been proven in studies to remove 120 clinically-relevant pathogens as well as the toxic products they release, known as Pathogen Associated Molecular Patterns (PAMPs), which trigger the inflammatory cascade that leads to sepsis.
“The holy grail will be direct capture and concentration from the blood, and identification using mass spectrometry, without the small amount of growth we had to do in the GOSH study.”
Michael Super
At this point, Ingber and Super had the capability to cleanse the blood of patients before the inflammatory cascade reached a tipping point and became full-blown sepsis, but they couldn’t identify the pathogen that caused that cascade. To do this, a diagnostic was required, but with the available methods taking at least a day, Ingber and Super had to find a way to decrease the time dramatically. Luckily, Darpa was on board with this idea too and in a subsequent project they funded the development of a diagnostic. “We had this great capture mechanism, but very poor identification,” says Super. “We can identify best with PCR if we know what we’re looking for, but the one that’s really good and fits in well with clinical practice is mass spectrometry, specifically MALDI-TOF mass spectrometry.” Matrix-assisted laser desorption-ionisation time-of-flight, or MALDI-TOF, is a type of mass spectrometry already used to help diagnose pathogens in systems like the Bruker Sepsityper kit, but the current method of centrifugation proved less accurate than combining the detection capability of the Sepsityper with FcMBL-coated beads. “It didn’t, for example, bind well to fungi or gram-positive [bacteria],” says Super. “It binds well to gramnegatives, whereas with the FcMBL technology, we were able to see all three.” Another important advantage was that the superior binding capability of FcMBL meant it could detect pathogens in lower quantities of blood.
This was a key part of the study, which was done in collaboration with Great Ormond Street Hospital for Children (GOSH). “It’s incredibly important in paediatrics because we find that you can’t get enough blood for the current blood cultures,” says Super.
Holy grail
The clinical value of identifying the pathogen(s) in the blood of a patient with sepsis isn’t hard to imagine; with a specific target, or potentially a group of targets, a physician can apply the correct antibiotic, antiviral, or antifungal medication, rather than taking the scattergun approach of broad-spectrum antibiotics. This is especially important, according to Super, given that cases of sepsis caused by fungal infection are on the rise. “In the time that I’ve been in this field, fungi have gone from the forgotten child to suddenly one of the major killers in ICUs,” he says.
Ingber and Super have achieved a great deal so far with FcMBL, with both the dialysis-like sepsis treatment device and the companion diagnostic licensed to BOA Biomedical, a Harvard spinout start-up company affiliated with technology investment and development company Miraki Innovation. But Super sees the next step as the most important for the clinic. “The holy grail will be direct capture and concentration from the blood, and identification using mass spectrometry, without the small amount of growth we had to do here [in the GOSH study],” he says. “If we could take the filter after the blood has been passed through it and extract the pathogens that were on it, then run those through a diagnostic, that to me is the next step. In fact, I feel we’re already there, we just haven’t done the study yet.”