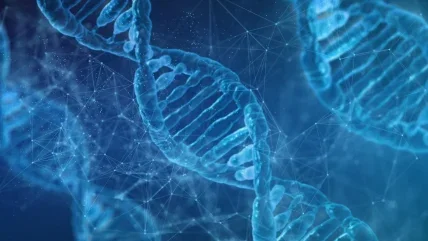
In 1700, Nicolas Andry made a remarkable claim: in a book published in English as An Account of the Breeding of Worms in Human Bodies, the French scientist argued that these eponymous ‘worms’ caused smallpox and other diseases. In the event, Andry was wrong: what his primitive microscope had spotted were microorganisms, not the virus that blighted faces all over Enlightenment Europe. All the same, Andry’s intuition would soon revolutionise medical science. Far from being caused by unbalanced humours, or else some miasma in a swamp, doctors would soon hunt for the causes of infections in microorganisms – not quite worms but living organisms all the same.
From these unsure beginnings, identifying the microorganisms behind diseases has become a staple task for medical researchers everywhere. According to work by The Insight Partners, to give one example, the ‘microbial identification methods’ market is expected to reach about $8bn by 2030: about double what it was in 2022. Nor is this bounding growth surprising. Allowing scientists to target treatment to specific microbes, understanding the root causes of infections lessens our reliance on broad-spectrum antibiotics, along the way stifling microbial resistance. That outcomes improve dramatically is obviously another advantage.
Whatever the sector’s financial strengths, however, the sector continues to face challenges. Though doctors now boast plenty of tools to identify common infections, like staphylococcus or the common flu, new or unusual pathogens have typically been far tougher to spot. That inevitably makes successful treatment much harder, even as time and money are spent on less effective alternatives. Yet, as so often in the clinical care space, new technology could soon consign these challenges to the same dustbin as Nicolas Andry’s ‘worms’. Known as clinical metagenomic next-generation sequencing (mNGS), this groundbreaking approach allows researchers to rapidly determine the microbe responsible for a particular illness, a godsend for patients and medical administrators alike. Not, of course, that the machines can do it all themselves – metagenomics can only really succeed if used in coordination with the human expertise of doctors and nurses.
Give it to me straight?
The medical calendar has regular rhythms. Every autumn, the flu rears its yearly head. Strep throat, for its part, tends to be more common in the winter and spring. While dispiriting from a medical perspective, this seasonality does have its uses. When it comes to common illnesses, after all, doctors can dovetail past experience and current symptoms to guess at a cause. That’s echoed by a plethora of diagnostic tests. When it comes to influenza, for example, some molecular tests offer results in as little as 15 minutes, even as accuracy hovers around the 95% mark.
But what happens if a patient arrives with unusual symptoms, ones that can’t easily be explained by flu or strep throat? For Dr Anne Jamet – head of mNGS at the Necker-Enfants Malades Hospital (AP-HP) in Paris, and a researcher at the Institut Necker-Enfants Malades – this can quickly cause difficulties. “Targeted research is limited by prior knowledge,” Jamet says, “making it unusable for emerging infectious diseases with new pathogens, which can also evolve rapidly.” Fair enough: if they don’t know what they’re looking for, researchers obviously can’t develop tests for specific pathogens. And even when they do have more information, traditional detection methods come with other problems. One tactic involves isolating and cultivating microorganisms, before testing for pathogens. But this process can sometimes take weeks, even as prior exposure to antibiotics can impair results.
“Targeted research is limited by prior knowledge, making it unusable for emerging infectious diseases with new pathogens, which can also evolve rapidly.”
Dr Anne Jamet
These technical irritations can lead to serious practical consequences. As Jamet explains, not knowing the specific cause of a disease can force doctors to “open the umbrella” – and ply patients with various drugs hoping they stick. Though this can succeed in the short term, it can make the pathogen more resistant to drugs later, even as they become more transmittable. There are other consequences too. “If infection is not properly treated, it is likely to be associated with prolonged illness and increased mortality rates,” Jamet warns, adding that longer hospital stays also mean more costs. Dr Jessica Galloway-Peña makes a similar point. Though she concedes the price of misdiagnosed infections is hard to quantify, the Texas A&M University professor stresses that misidentification of illnesses such as sepsis can be fatal. It hardly helps that antimicrobial resistance costs $55bn a year in the US alone.
$55bn
The annual cost of antimicrobial resistance in the US.
NIH
Next (patho)gen
Over the past few years, a solution to these varied frustrations has emerged. As the name implies, clinical metagenomic next-generation sequencing differs significantly from older forms of pathogen detection. Rather than growing the organism in a lab, a testing sample can instead be taken directly from a patient’s stool or urine. From there, scientists extract the relevant genetic material, in a process called library preparation, before testing it in a sequencing machine. Just a few hours later, millions of sequences (or ‘reads’) are available for analysis, something typically done by specialised bioinformatics software.
“This is important for rare, and novel pathogens with new aetiology.”
Dr Jessica Galloway-Peña
Certainly, that’s far quicker and simpler than cultivating a sample – but what makes mNGS special is its flexibility. Rather than needing prior knowledge about what to look for, the technology allows researchers to examine all the microbial genomes present in any given sample – both familiar examples and totally new arrivals. As Galloway-Peña says: “This is important for rare, and novel pathogens with new aetiology.” Jamet, for her part, has proof of this in practice. Over three years from 2019, in partnership with colleagues at the Institut Pasteur’s Pathogen Discovery Laboratory, she used mNGS technology to analyse over 700 samples. The first comprehensive study of its kind in France, it involved subjects from various backgrounds. The results were striking. Proving what Jamet calls the “high positivity rate” of tissue analysis, the study underlined the strengths of mNGS’s non-targeted approach – especially among immunocompromised patients suffering otherwise mysterious ailments.
700+
The number of samples taken for mNGS analysis by Jamet and her team.
Institut Pasteur
The rising popularity of mNGS is clear financially too. As research by CoherentMI found, the global metagenomic sequencing market is predicted to hit the $4.3bn mark by the end of the decade, along the way enjoying a remarkable CAGR of 17.6%. All the same, it’d be wrong to imagine mNGS as a panacea. While it can helpfully pinpoint relevant pathogens, doctors can’t ignore a patient’s symptoms and biological signs either. No wonder Jamet notes that after the reads have been analysed by bioinformatics software, clinical microbiologists must still interpret the results – before passing on their findings to clinicians. “In clinical practice,” she stresses, “diagnosing an infection is a real team effort.”
$2,250
The average cost of a single mNGS test.
ADLM
A needle in a haystack
Other limitations of mNGS can’t be solved by collaboration. Some are technological. Though it’s adept at analysing the genetic material present in a sample, for example, that encompasses both pathogens and regular human DNA. “The problem,” Jamet says, “is that the patient’s genetic material can be much more abundant than that of the pathogens, so it can be difficult to find a needle in a haystack.” In a similar vein, mNGS can struggle to detect mycobacteria and fungi, while it also risks missing low-abundance pathogens.
Other factors, from how human nucleic acids are extracted to which sequencing reagents are chosen, can potentially distort the results of metagenomics too. As Galloway-Peña adds, different referencing databases can impact outcomes too. Bolstering the technology’s standardisation is therefore a must, as is ensuring platforms are accessible. Though she concedes that sequencing instruments are “widely available” across medical life, Jamet notes that “there are as yet no standardised, user-friendly solutions for adopting mNGS in clinical laboratories lacking local bioinformatics expertise.” Cost is undoubtedly another problem: a single test can cost $2,000–2,500.
Not that the situation is hopeless. For one thing, wider adoption promises to nudge prices down, with resource pooling one obvious tactic. More generally, both Jamet and Galloway-Peña are confident that the scientific foibles of mNGS could soon be ironed out. As the French expert says, the “continuing refinement of bioinformatics techniques and algorithms” may shortly allow researchers to identify fungi alongside viruses and other pathogens. Galloway-Peña seems similarly optimistic, especially when it comes to standardisation. “If,” she says, “we can actually figure out a way to decrease the high variability, and have FDA-approved platforms with use and reporting guidelines,” then she believes mNGS could be an excellent tool to diagnose patients, and identify new threats. Considering how revolutionary metagenomics could soon be, you have to hope she’s right.