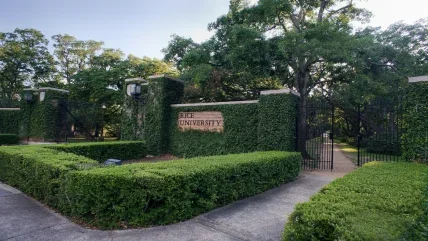
MRI scanners image the body’s interior by briefly aligning the nuclei of hydrogen atoms and measuring how long it takes the nuclei to relax to their resting state. Relaxation properties vary by tissue, and by repeatedly aligning nuclei and measuring relaxation times, an MRI scanner builds a detailed image of the body’s organs, tissues and structures.
Within this process, contrast agents improve scan resolution by increasing the relaxation rate of particles. Radiologists can weigh the results of an MRI, making specific tissues appear either brighter or darker by varying the conditions of the test. Two weighting techniques, named T1 and T2, are commonly used.
Gadolinium chelates revolutionised MRI testing when they were introduced in the late 1980s, and have been used more than 400 million times in the years since. Though gadolinium is a toxic metal, the chelating process covers each gadolinium ion with an organic wrap that reduces exposure and allows the drug to pass from the body via urination within a few hours
In 2013, however, Japanese scientists made the discovery that gadolinium from contrast agents had accumulated in the brains of some patients, and subsequent studies found similiar deposits in bones and other organs.
Experts previously believed gadolinium contrast material could not cross the blood-brain barrier, the semipermeable membrane that selectively filters materials from the bloodstream from entering extracellular fluid in the brain, as well as the central nervous system. Studies soon followed with attempts to gauge the risks the scenario posed.
Still, very little is known about the health effects of gadolinium that is retained in the brain. A major study, presented at the annual meeting of the Radiological Society of North America late last year, found no evidence that accumulation of gadolinium in the brain speeds cognitive decline.
Independent risk factors
For this study, lead author Dr Robert J McDonald, neuroradiologist at the Mayo Clinic, and his colleagues set out to identify the neurotoxic potential of intracranial gadolinium deposition following intravenous administration of gadolinium-based contrast agents during MRI.
The researchers used the Mayo Clinic Study of Aging (MCSA), the world’s largest prospective population-based cohort on ageing, to study the effects of gadolinium exposure on neurologic and neurocognitive function.
All MCSA participants underwent extensive neurologic evaluation and neuropsychological testing at baseline and 15-month follow-up intervals. Neurologic and neurocognitive scores were compared using standard methods between MCSA patients with no history of prior gadolinium exposure and those who underwent prior MRI with gadolinium-based contrast agents. Progression from normal cognitive status to mild cognitive impairment and dementia was assessed using multistate Markov model analysis.
The study included 4,261 cognitively normal men and women aged 50–90, with a mean age of 72. Mean length of study participation was three years and seven months. Of the 4,261 participants, 1,092 (25.6%) had received one or more doses of gadolinium-based contrast agents, with at least one participant receiving as many as 28 prior doses. Median time since first gadolinium exposure was five and a half years.
After adjusting for age, sex, education level, baseline neurocognitive performance and other mitigating factors, gadolinium exposure was not a significant predictor of cognitive decline, dementia, diminished neuropsychological performance or diminished motor performance. No dose-related effects were observed among these metrics. The conclusion was in – gadolinium exposure was not a risk factor in the rate of cognitive decline from normal cognitive status to dementia in this study group.
“There is concern over the safety of gadolinium-based contrast agents, particularly relating to gadolinium retention in the brain and other tissues,” McDonald acknowledged upon publication. “This study provides useful data that at the reasonable doses 95% of the population is likely to receive in their lifetime, there is no evidence at this point that gadolinium retention in the brain is associated with adverse clinical outcomes.”
Despite these findings, the safety of gadolinium-based contrast agents used in magnetic resonance imaging remains a major source of debate in the medical and public spheres. Just one month after the report’s release, FDA launched a requirement for drug makers to add warnings to the medication guides for eight widely used gadolinium-based contrast agents.
– Naomi Halas, lead researcher
Chock-full of iron
Within this environment, research into alternative contrast agents has gathered pace, and Rice University nanoscientists claim to have developed a method for loading iron inside nanoparticles to create MRI contrast agents that outperform gadolinium chelates.
“The possibility of eliminating gadolinium exposure and getting a two-fold improvement in T1 MRI contrast performance is going to intrigue radiologists,” says Rice’s Naomi Halas, the lead researcher on the project. “When they hear we have done this with iron, I expect they will be very surprised.”
While iron-based contrast agents are frequently employed for T2 scans, there are few clinically available alternatives to gadolinium for T1 tests.
“Iron chelates aren’t new,” Halas acknowledges. “It’s widely believed they are wholly impractical for T1 contrast, but this study is a perfect illustration of how differently things can behave when you engineer at the nanoscale.”
Halas and colleagues from Rice, as well as the University of Texas Anderson Cancer Center, published their findings in a paper made available in the American Chemical Society journal, ACS Nano. In the study, the collective created a modified version of nanomatryoshkas, concentric layered nanoparticles that draw their name from Russian nesting dolls.
Nanomatryoshkas and nanoshells, another layered nanoparticle Halas invented at Rice more than 20 years ago, are about 20 times smaller than a red blood cell and made up of layers of conductive metal and non-conductive silica. By varying the thickness of the layers, Halas’s team tuned the particles to interact with specific wavelengths of light. For instance, nanoshells and nanomatryoshkas can convert otherwise harmless near-infrared light to heat. This localised, intense heating has been used to destroy cancer in several trials of nanoshells, including an ongoing trial for the treatment of prostate cancer.
The new study is the latest chapter in Halas’s efforts to create lightactivated nanoparticles with a combination of therapeutic and diagnostic features. These theranostic particles could allow clinicians to diagnose and treat cancer in the same office or hospital visit.
Luke Henderson, a Rice graduate student and lead author of the ACS Nano paper said, “If clinicians could visualise the particles through some sort of imaging, therapy could be faster and more effective. For example, imagine a scenario where a scan is performed to verify the size and placement of the tumour, heat is then generated to treat the tumour and another scan follows to verify that the entire tumour was destroyed.”
When Henderson, a chemist, joined Halas’s Laboratory for Nanophotonics in 2016, Halas’s team had already shown it could add fluorescent dyes to nanomatryoshkas to make them visible in diagnostic scans. Work was under way on a study published in 2017 that showed gadolinium chelates could be embedded in the silica layer for MRI contrast.
“In the earlier work with gadolinium, we noticed that the nanomatryoshka design enhanced the relaxivities of the embedded gadolinium chelates,” Henderson said. “At the same time, we were hearing more calls from the medical community for alternatives to gadolinium, and we decided to try iron chelates and see if we got the same sort of enhancement.”
– Luke Henderson, lead author of ACS Nano paper
A nice surprise
The results surprised everyone. Not only was Henderson able to boost the relaxivities for iron, he was able to load about four times more iron into each nanomatryoshkas. That allowed the iron-laden nanomatryoshkas to perform twice as well as clinically available gadolinium chelates.
Henderson found a useful way to change the type of metal that was loaded. By adding unloaded chelate molecules to the silica first, he found he could load metal by soaking the particles in a bath of metal salts. By changing the metals in the bath, he found he could easily load different paramagnetic ions, including manganese, into the nanomatryoshkas.
After the metal ions were loaded into the silica, the final layer of the nanomatryoshka, the outer gold shell, was added. The shell, which is vital or plasmonics, serves as a barrier to prevent ion leeching. Henderson said the gold barrier had a secondary benefit for the fluorescent dyes he added for dual-mode diagnostics.
“All fluorescent dyes are subject to photobleaching, which means they fade over time and eventually won’t give off a measureable signal,” Henderson said. “Even if you freeze them, which slows down bleaching, they typically don’t last more than a couple of weeks. I was looking at an old sample of nanomatryoshkas that had been in the fridge for months, and I found they were still fluorescing quite well. When we looked more closely at this we found the dyes were about 23 times more stable when they were inside the nanomatryoshkas.”