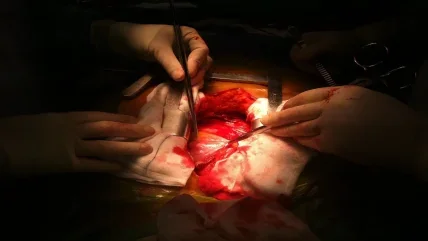
A few years ago, the idea of using 3D-printing technology for heart surgery would have drawn blank stares from most. But now, the Cleveland Clinic Abu Dhabi – a multispeciality hospital on Al Maryah Island – is seeing what it can do to monitor and improve wound care.
The clinic is part of the Mubadala network and is an extension of US-based Cleveland Clinic. It has been specifically designed to address a range of complex and critical care requirements that are unique to Abu Dhabi’s population. The physician-led medical facility, which is served by US and European board-certified, or equivalent, professionals, is also using cutting-edge 3D-printing technology in its cardiac procedures to customise care for its patients.
Currently, the technology is used at the institute for certain heart surgeries and non-coronary catheter-based interventions, such as valve and device implants, and surgery for congenital heart diseases. The facility is exploring integrating 3D-printing technology into other applications within the facility, as well as the radiology department.
This procedure is well integrated: after a CT scan of the heart is taken at the hospital, the image is sent to a third party where engineers develop a 3D model using the scan. This is then sent back to the clinic’s physicians.
Globally, the use of 3D printing in medicine has been on the rise over the past few years, and it is commonly used to design tissues and organs, and create customised prosthetics and implants. It also helps in pharmaceutical research.
Practical Patient Care Middle East speaks to Dr Thomas Bartel, section head of interventional cardiology at the Heart & Vascular Institute of the Cleveland Clinic Abu Dhabi, about the use of 3D printing in cardiovascular medicine and cardiac surgery.
His role also requires him to lead the institution’s Program for Management of Structural Heart Diseases. “Altogether, that implies catheter-based diagnoses and therapy in patients with coronary heart disease, valvular and other structural disorders; for example, aortic stenosis, mitral regurgitation, interatrial communications, paravalvular leaks after valve replacement, and so on,” he says.
“In our institution, decision-making is based on the ‘heart-team approach’. That means all decisions on the management of complex heart disease are made in teams consisting of heart surgeons, invasive and non-invasive cardiologists, radiologists and colleagues from other disciplines as needed.”
Practical Patient Care Middle East: Why is new technology like this valuable in the world of cardiology, and what were the reasons behind its necessity in the first place?
Dr Thomas Bartel: With the high complexity of cardiac conditions on the one hand, and the rising number of patients with comorbidities on the other, current surgical and interventional therapies need individualisation. That is why procedures require advanced peri-procedural imaging, and sometimes even tactile testing, to become as safe, expedient and well tolerated for patients as possible.
Nowadays, peri-procedural imaging is based on volume rendering from 3D data sets obtained from cardiac computed tomography, MRI and 3D echocardiographic studies being portrayed on a two-dimensional monitor. Although these techniques are helpful, conventional 3D imaging alone is not as effective as having a physical model that is identical to patient’s anatomy, particularly prior to sophisticated interventional and surgical therapeutic approaches.
Can you explain the technology, and how it allows the development for a 3D model using the scan, particularly the procedures that surround this?
The workflow through to 3D printouts starts from conventionally obtained 3D data sets, in which objects of interest are selected and separated. This process is called segmentation and is done under the Digital Imaging and Communications in Medicine (DICOM) standard. However, DICOM image files cannot be used by 3D printers and, therefore, have to be converted into another standard called standard tessellation language (STL). This format states that the surfaces of anatomic structures are composed of small triangles that fit together like a spatial puzzle.
Before 3D printing can start, STL models have to be post-processed accordingly. Candidates for peri-interventional 3D printing are those patients who present highly individual pathology and significant additional intra-operative risks. If anatomic features and spatial relation to the surrounding structures are critical for selecting optimal approach and evaluation of procedural results, then 3D printing is capable of helping with optimised planning and the simulation of a particular procedure by direct use of the model.
This model is used for specific heart surgeries and non-coronary catheter-based interventions, including valve and device implants, as well as surgery for congenital heart diseases. What are the limitations of developing procedures for its use, and where would you like to extend the use of this particular technology?
Indeed, models are used for these procedures. I cannot see any extension of indications at this point in time. A major issue we have to deal with is the lack of evidence; up to now, there has been no randomised data available to the warrant expenses of 3D printing. The next step to take is evaluation following phantom studies and clinical trials. At present, we don´t
know whether the quantitative dimensions that are reflected by the models really correspond to the true ones in the human body. So we are still in an early phase prior to extensive clinical use. It is also important to note that the costs are not yet covered by health insurers.
How does using 3D-printing technology in cardiac procedures help to customise care for patients?
Modelling can help to accommodate devices for pathology; for example, in a device closure of the left atrial appendage or atrial septal defect with atypical shape and anatomy, devices can be tested for fit accuracy. Catheters can also be tested and preselected to optimise and shorten approaches to target regions; for instance, in radio frequency ablation procedures. These procedures often take hours because the target region is usually not easy to approach precisely.
What benefits to surgical planning and procedures does it offer, and how does this help in terms of wound care?
As far as we know, the method is not eligible to directly help in wound care or other immediate post-operative care. The point is that finding the optimal surgical access can be extremely challenging in pre-operated patients and those with cardiac malposition, congenital or other structural heart disease. Scenarios like these are hard to manage, even for experienced surgeons. Modelling may help to keep access wounds as small as possible, and it may also assist during follow ups after device implantation, in terms of preserving the immediate post-operative result. In particular, 3D printing enables surgeons to choose the most atraumatic way of surgery. Model -based testing and the selection of surgical instruments could help to shorten operation time.
You mention in the press that if any concerns arise during the operation, “we can use the 3D-organ replica to determine the best course of action in real time”. Can you elaborate on this?
This applies to troubleshooting. Limited intra-operative visibility to the target region is inherent to many new procedures that are currently under development. In that regard, we hope for improved intra-procedural orientation from 3D printing.
Are there any downsides to it, and where do you see this technology going?
Using this technology is far from becoming routine. For the time being, it will be reserved for complex procedures where standard approaches do not apply. Subsequent development and penetration of 3D printing into daily practice depends predominantly on the demonstration of verifiable benefits for patients. In the era of evidence-based medicine, manufacturers and users have to evaluate the technique according to the standards that apply for medical trials before introducing it into common use.
Another group of techniques called 3D bioprinting are thought to be able to build engineered-tissue constructs with complex and hierarchical structures, as well as mechanical and biological heterogeneity. New bionic materials include cell suspensions, cell-laden hydrogels, decellularised matrix components, microcarriers that offer particular surface areas for quick cell attachment and scaffold-free cell spheroids, which enable more mature tissue constructs. However, despite great progress in research, there are still many challenges that prevent the transition of these techniques to clinical applications.